Investigating a Novel Bacterial Form for Biological Computers
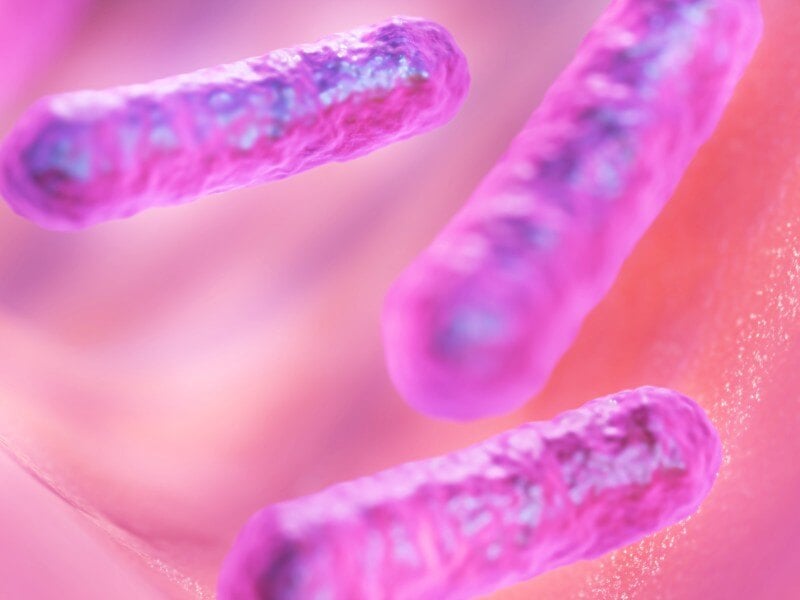
All of modern computing relies on a very simple building block. The inverter or NOT gate simply takes an input, 1 or 0, on or off, and reverses it. Combining the NOT gate with another, the OR gate, results in 'functional completeness': the ability to create all other logical functions using only these constituents.
The simplicity of computing's basic ingredients has led researchers to attempt to recapitulate computers with biology, sometimes towards therapeutic endpoints. This idea comes under the broad umbrella of synthetic biology, which uses engineering principles to design biological systems for useful purposes.
Traditionally, the principle that powers 'biological computers' has been the genetic programming of DNA sequences in living cells (methods such as Cello), but the size of these genetic programs is limited by the linearity of genetic code.
Therefore, a study from a group of researchers at University College London has demonstrated a design for biological computation which instead uses bacteria: "computational communities of microbes have been developed in liquid culture. This allows the division of a complex function into smaller, isolated modules that can be programmed into different populations and integrated using inter-population communication."
"The limitations of monoculture computing and distributed communities in liquid culture can be overcome by producing logic gates based on spatially arranged, communicating bacterial colonies in solid culture."
The paper, published in Nature Communications, outlines how simple functions can be encoded into spatially discrete colonies of bacteria which can communicate with one another. From this, these colonies can be built on-top of each other to program more complex functions.
The paper states that their method is able to "produce modular, easily programmable bacterial computers."
Rather than using genetic engineering, the programming of these biological computers is encoded using morphogen gradients. Bacterial cells either send or receive signals using gradients of diffused molecules, and the programming of the function is determined by the position of the receiver in relation to the sending cells.
It works like this: each input produces a diffusion field of molecules as a radial gradient. The concentration of these molecules is higher nearer the input, and lower further away. Two inputs, A and B, spaced strategically, will have a space between them that has a high concentration of molecules if either one or both of the inputs are on.
How does this produce a logic gate? It depends how you detect the concentration of molecules. By probing this space in between the two inputs, A and B, and detecting only high concentrations of molecules (what the paper describes as a 'highpass filter'), this probe will act as an OR gate. If A is on, the probe will detect a high concentration; if B is on, likewise; the same will be true if both are on.
Furthermore, by probing this same section, but instead only set out to detect low concentrations of molecules (called a 'lowpass filter'), the configuration effectively acts as a NOR gate. If A is on, the concentration in the middle will be too high for the probe; if B is on, likewise; if A and B are on, the concentration will be far too high; only when both A and B are off will the concentration of molecules be low enough to alert the probe. Thus, a NOR gate.
From this basic principle, all manner of logic gates can be made based on where and how the concentration of molecules is detected, either highpass, lowpass, or bandpass (where only moderate concentrations are detected).
The aforementioned probe, in the case of the experiment is a receiver cell which can produce a fluorescent output based on their programming. This modulates the modal diffusion gradient into a binary 'on or off' signal, which can be connected to other logical modules elsewhere.
The paper says: "Our approach will open up ways to perform biological computation, with applications in bioengineering, biomaterials and biosensing. Ultimately, these computational bacterial communities will help us explore information processing in natural biological systems."