Overcoming the Challenges of Precision Genome Editing
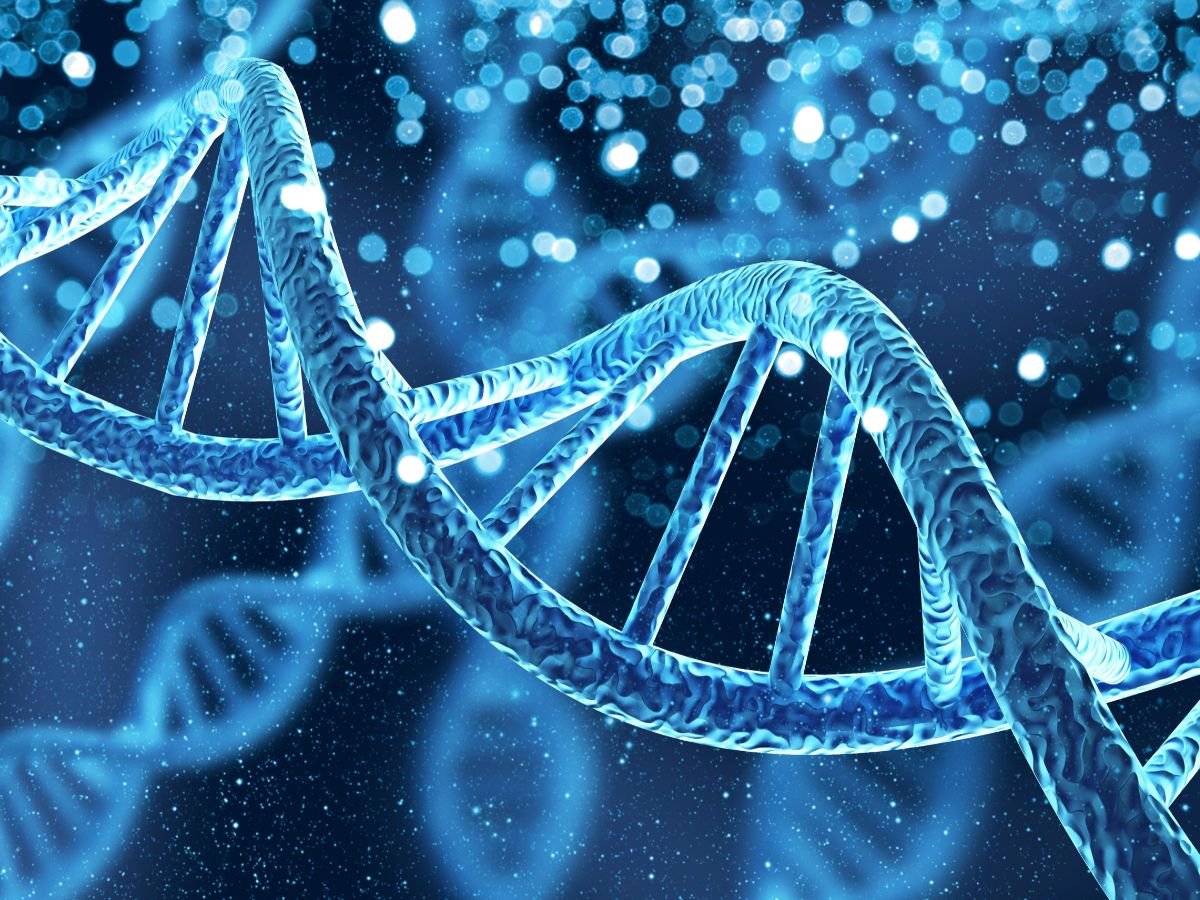
Precision genome editing refers to the ability to directly modify and change DNA. Genome editing is made possible by DNA-binding platforms and technologies that locate target-specific genomic sequences and associated biochemical activity. Examples of such technologies includes CRISPR–Cas9, zinc fingers, and base and prime editing, to name a few.
The need for precision genome editing can be largely attributed to the improved detection and characterisation of chronic and genetic disorders, which has expanded the need for research and development in the genomics field. Similarly, continuous advances in gene therapy and the broadening therapeutic applications of genome editing tools in the pharmaceutical, biotechnology, and agricultural sectors have resulted in rapid market growth.
In 2020, the global gene editing market was valued at 5.2 billion USD and is expected to increase to 18.5 billion in 2028. With a compound annual growth rate (CAGR) of 17.2% for the forecasted period, it's no wonder that precision genome editing was one of the trending topics of conversation and research presentation at our annual Gene Therapy Development &Manufacturing: In-Person event held in June 2022.
- Understanding CRISPR: When to Cut and When to Inhibit?
- Refining Analytics for Gene Delivery Systems
- Inciting Rapid Standardisation in Cell and Gene Therapy Manufacturing
One of our most engaging sessions on the topic was a roundtable discussion hosted by Patrick Harrison, Senior Lecturer at the University College Cork, which looked at ‘Overcoming Challenges Facing Precision Genome Editing.' Harrison was joined by other experts in the field: Paola Dama, Research Fellow at the University of Sussex; and Benjamin Taylor, Director of Cell Biology &Immunology at AstraZeneca.
In this article, we look at some of the key insights divulged and lessons learnt from this stimulating roundtable and discussion.
Delivery Design Dilemma
According to Harrison, one of the utmost considerations in precision genome editing is to “figure out whether the delivery method shapes the choice of your target disease or not.” Harrison spoke of his experience with CAR T cells and contrasted his research group's focus on cystic fibrosis, with treating diseases that are typically easier to deliver to. “With retinal disorders, there is relatively easy access to the eye, and then for ex vivo studies using CAR T cells you can efficiently harvest the cells from an affected individual, modify them, and then safely infuse them back,” he continued.
The broadening therapeutic applications of genome editing tools in the pharmaceutical, biotechnology, and agricultural sectors have resulted in rapid market growth.
With such diseases there is a level of control in terms of delivery efficiency for the patient. This approach offers a more successful alternative to what Taylor describes as “starting with the disease first and trying to fix the delivery problem later.” The latter can result in significant bottlenecks during administration. “For many of these projects you get stuck in the delivery stage - you can treat the disease, but you cannot deliver the treatment,” he continued.
The Intricacies of Precision Genome Editing
The traditional editing strategies for gene therapy included performing a double-stranded break followed by a homology-directed repair to precisely correct a disease-causing mutation, and the exploitation of targeted knock-out of a gene, in some cases to reduce the expression of a pathogenic gene. Over the past five years, however, base and prime editing strategies have started to trend within the industry. Whilst these have proved highly efficient, there remain limitations on various targets.
Improvements are now underway to ensure sufficient target opportunities for these editing tools. Prime editing, in particular, holds great promise in increasing target availability. One way to do so is to deliver via the editor and guide as RNA molecules, which removes DNA requirements and provides a potentially safer editing tool that reduces the chances random integration of delivery components. A further strategy of improvement has been making a nick on one strand which significantly reduces the levels of indels and potential translocations.
Strategies to Optimise Precision Genome Editing Technology
There is no denying that genome editing techniques and technologies have already come a long way in the recent past. But there is still a way to go before the industry can fully realise an advanced approach to such tools. Dama believes that greater care must be taken in deciding “which technology is preferable for use as achieving an optimised editing result largely depends on your targets and their range.”
Over the past five years, base and prime editing strategies have started to trend within the industry.
Speaking of her experience at The University of Sussex, Dama provided the example of relying on a pooled library screening process to pair guide RNA. “The pair guide RNA allows us to target the 3'UTR of all of the many hundreds of targets of a particular micro-RNA that we know is important in the tumorigenesis of pancreatic cancer”, she explained. “This way, we can provide two guides that allow us to conduct appropriate deletion and avert disruption at the sequence of interest. In addition, the Dual gRNA pool library screening is a magnificent tool to study non-coding RNA.”
Taylor, on the other hand, attests to the promise of base editing technology, which he sees as continuing to “work beautifully and efficiently on certain targets.” Areas of innovation will need to address off-target functionality. “There has already been a lot of work done on guide RNA dependent off-targets for base editors, where mutations occur wherever CRISPR-associated (Cas) proteins sit in the genome,” Taylor confirmed. What isn't yet fully understood is the occurrence of guide RNA independent deamination. Deamination in this case refers to the specific removal of the amino group of cytidine to form uracil in the DNA.
As Taylor puts it, “we need to find solid answers about these deaminase enzymes that float around the genome and drive mutations that could potentially lead to cancer”. Whilst many studies highlight these are low frequency, this activity could be below the threshold of our detection methods, so further study is necessary for confirmation of this fact. This is because there may be mutations that remain undetectable at this present time.
Final Thoughts
Other development areas for precision genome editing include refining prime editing tools for better clinical application. Both Taylor and Harrison agreed that “while it looks good on paper, it has proved challenging to implement efficiently in the lab, but a number of recent studies suggest several ways to boost efficiency.” Some of the opportunities for achieving this may also include implementing computational tools to identify and enhance the efficiency of correction of disease-relevant mutations.
Precision genome editing continues to present an exciting opportunity for the cell and gene therapy landscape. As innovation and evolution persists, we can expect to see great things from the robust and versatile platforms already in use. At Oxford Global we look forward to seeing just how the next generation of precision genome editing will empower scientists and researchers alike in their unfailing quest to unlock the future of therapeutics.
Want to find out more about the latest cell therapy news? Register now for Oxford Global‘s Cell UK: In-Person event taking place on 7-8 November 2022 to advance your understanding of cell-based products to ensure clinical and commercial success.