3D Cell Model Approaches for Disease Monitoring and Detection
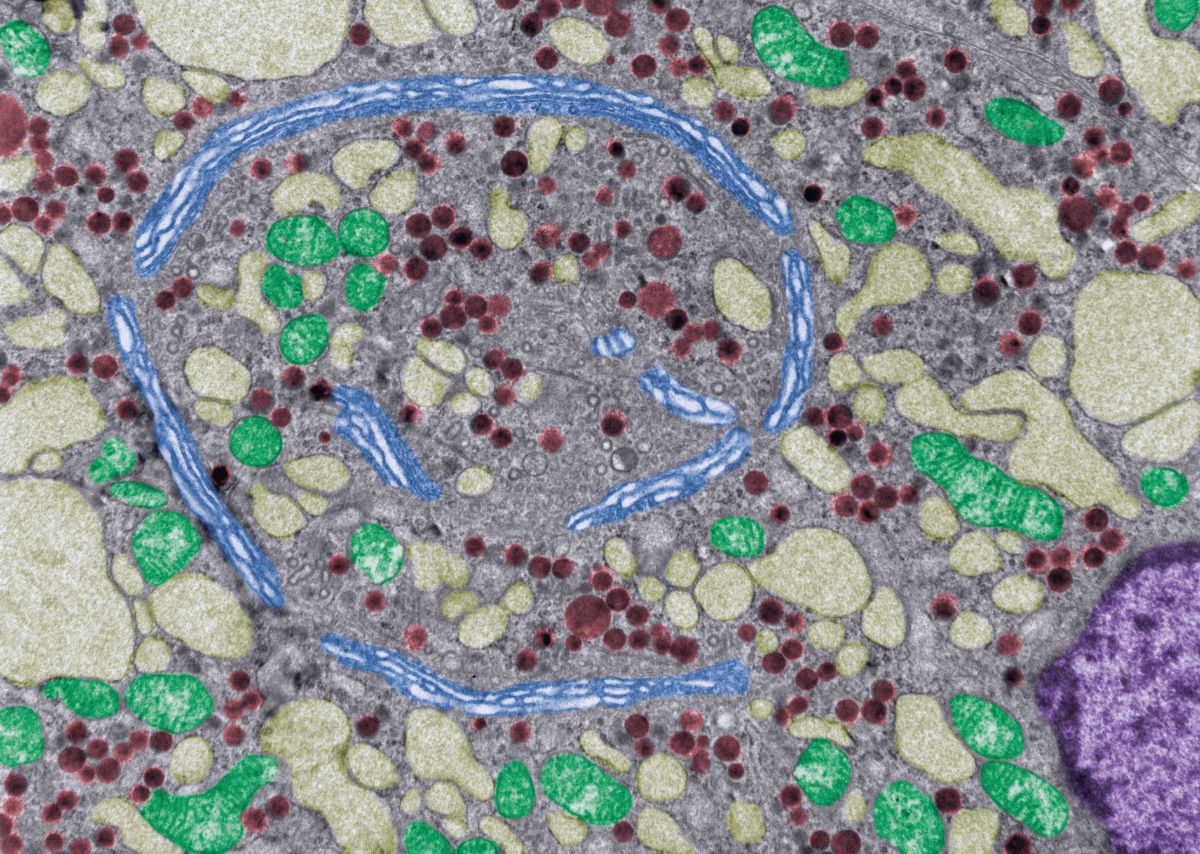
Cancers and cardiovascular diseases are among the leading causes of death worldwide. 3D cell model approaches for disease research and tumour monitoring have the potential to form an integral part of the overall developmental process for new therapeutics and developmental drugs. For cardiac disorders in particular, most treatments are palliative rather than curative. With both, the underlying mechanisms dictating the spread and behaviour of the disease can vary wildly from patient to patient.
- The broad uses of 3D cell cultures across disease modelling and toxicology
- Cardiff University builds Lego 3D bioprinter capable of synthesising human skin
- Constructing in vitro models capable of replicating body tissue
As a result, developing an accurate means of modelling these diseases could fundamentally change efforts to provide treatment. Two presentations from 3D Cell Culture 2023 explored some cutting-edge approaches to 3D cell modelling to recreate the behaviour and spread of diseases in vivo, with consideration given to how this may reinforce future treatment approaches.
3D Cell Models for Safe Drug Development and Disease Treatment
Cardiovascular safety is a core focus across the pharmaceutical sphere – regardless of the nature of the therapeutic being produced, ensuring patient safety is paramount. In the last decade, the generation of cardiac disease models based on human induced pluripotent stem cells (hiPSCs) has become commonplace, providing new opportunities for cardiac modelling. A common example of this approach in safety involves utilising hiPSC-derived cardiomyocytes to investigate heartbeat rate as a surrogate for changes in vivo.
In the context of drug development, a therapeutic's progress through the developmental pipeline predicates which assay will be selected. Early in the development pipeline, more emphasis is placed on throughput, as there are typically thousands of candidate molecules that developers and manufacturers need to risk assess. As developers move forward, they gain access to more complex models which enable investigation of mechanistic insights.
These models can then be worked in with general setup and development further down the pipeline with greater clinical relevance, incorporating aspects such as AI. One area many in the industry are looking to address at present is patient-centricity in models, particularly in the context of the expanding modality landscape.
Utilising Cardiovascular 3D Models and Other 3D Model Approaches
One of the biggest benefits to utilising 3D models in drug development is the inclusion of different cell types in modelling. Across multiple cell types, researchers can gain insights into communications between different cells, which will happen naturally in vivo. With these 3D models, researchers can begin to incorporate more patient-relevant cells and then swap them as appropriate. A key challenge associated with the drug development process concerns drug-induced cardiotoxicity: current in vitro models are limited in their ability to detect these liabilities. Subsequently, companies such as AstraZeneca are looking to improve model quality to access and recreate a broader spectrum of possibilities.
This improvement came in the newest iteration of the human myocardium model, which is composed of heterogeneous cell types. This model is used to predict cardiotoxicity by interpreting an integrated readout of three endpoints implicated in cardiac pathology and necrosis. These endpoints are mitochondria membrane potential, endoplasmic reticulum integrity, and ATP as a readout. This assay is now routinely used in the drug development pipeline at AstraZeneca to help provide insights into whether a compound is likely to have a structural cardiotoxic phenotype.
Biometric Tissue Models for Studying the Tumour-Stroma Interface
The stromal tissues of the body are extremely well-regulated, with a distinct tissue architecture in terms of their surface topography and stiffness. However, solid tumours – unregulated masses that grow amongst healthy normal stromal tissues – are more unpredictable in their behaviour. In situ cancers use the vasculature of the host to both survive and disseminate itself, facilitating growth and future metastasis. As such, a key focus in engineering a tumour model should also be on engineering the healthy stroma surrounding it, as the interactions between tumour and host tissue help the cancer to progress.
Umber Cheema is Professor in Bioengineering at the UCL Centre for 3D Models of Health and Disease, and Vice-Dean in Innovation and Enterprise for the Faculty of Medical Sciences. Her focus has been on generating and modelling 3D tissue for the analysis of cancer spread and development, with a particular focus on tumouroid models. These models are generated by engineering specific compartments for stromal cells and embedding in engineered cancer masses to investigate the interactions between the two components. Cancer cells can invade across the boundary between the engineered artificial cancer mass and into the engineered stroma.
“What is key to this technology is you can decide what extra-cellular matrix goes into your cancer mass and what goes into your surrounding stroma,” explained Cheema. From here, researchers can monitor the interactions between tumour and host tissue, recapitulating some of those properties in terms of stiffness. Tumouroids are fabricated via a plastic compression technique used to compress collagen hydrogels to generate dense, biomimetic collagen matrices.
Tumouroid Cell Production
Discussing the production of tumouroid modes, Cheema said that the process starts with setting tumour cells within 3D collagen hydrogels. “You set your cell embedded hydrogels for 15 minutes to allow for fibrillogenesis,” she explained. Afterwards, we apply plastic compression using absorbers to remove the excess fluid, leaving behind a collagen scaffold with increased cell and collagen density. “Over the last two decades we've tested the effect of plastic compression on approximately 35 different cell types, both primary and cell lines,” Cheema added. “None of the cells are adversely affected, they all survive this process.”
Once the model is complete, it can then be developed into a more complex tumouroid with an inner cancer mass in a surrounding stroma; alternatively, it can be left as a simple tumouroid for studying the interactions of tumour cells directly with the matrix. To develop a more complex tumouroid, the tumour mass is embedded in a fresh collagen hydrogel. “You basically set a stromal compartment around a cancer mass and then compress the compartmentalised model,” said Cheema. “What you end up with is a clearly-defined tumour-stroma boundary which is crucial for quantification of invasion.” This enables Cheema and her research team to observe cancer spread and behaviour and precisely quantify cancer behaviour.
One aspect of generic tumour property that Cheema was particularly keen to capture was stiffness, as there is ongoing research in the medical community into the degree to which stiffness promotes cancer invasion. “It's very difficult to do with an organoid culture, but with tumouroids you can see boundaries and you can quite clearly delineate the cell-matrix remodelling and cancer invasion,” she explained. Collagen models could be used to investigate correlations between cancer spread and tissue stiffness, as well as the considerations that may arise from this.
Future Approaches and Applications for 3D Cell Models
Across both approaches to 3D cell models, two central tenets are clear: consistency and reliability. Both also emphasise the importance of studying cellular behaviours and interactions in the broader context of the tissue that they inhabit. Maintaining this context in disease modelling is crucial for developing a comprehensive understanding of how different diseases behave.
A forester looking to assess the spread of disease between trees in a woodland would obviously benefit from conducting a detailed analysis of an oak tree with blight. However, their work would benefit much more from investigating how this oak interacted with the other trees surrounding it. The same principle holds true for today's 3D models: by looking at interactivity and other behaviours in more detail, researchers like Cheema are helping to pioneer the detection of disease and the development of new treatments.
Want to receive the latest industry announcements on cell manufacture and development? Sign up for our Cell series newsletter to get up-to-date news each month. If you'd like to know more about our upcoming 3D Cell Culture conference, visit our event website to download an agenda and register your interest.