Three Studies in 3D Stem Cell Culture
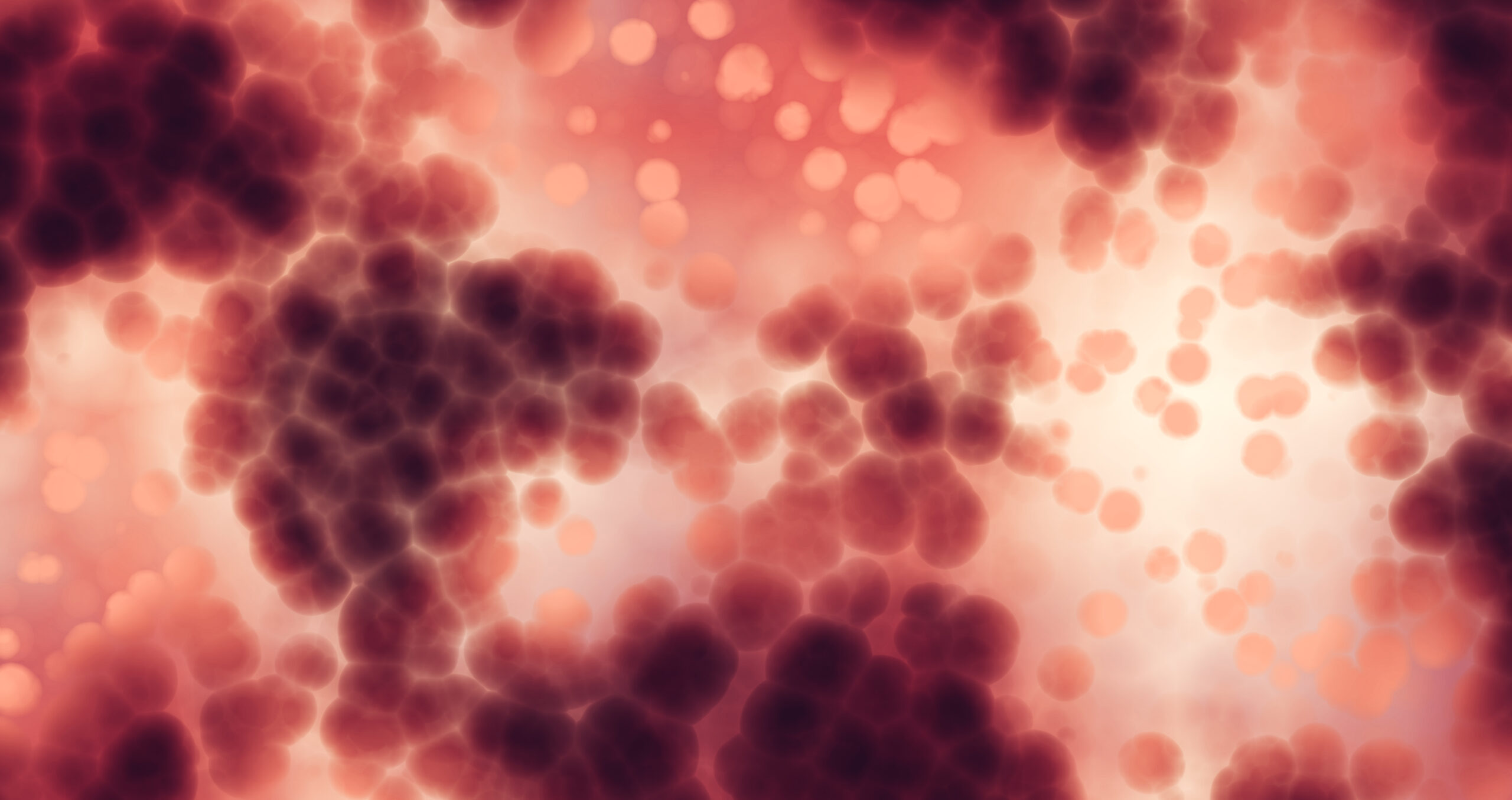
At Oxford Global's 2021 3D Cell Culture symposium, we were delighted to host an intriguing presentation from Dr Darius Widera, Associate Professor of Stem Cell Biology and Regenerative Medicine at the University of Reading. Dr Widera, whose presentation concerned the 3D culture of stem cells, discussed three hydrogels used as a scaffold for proliferating stem cells in three dimensions. In his presentation, Dr Widera looked at three case studies showcasing each hydrogel and took us through the data to assess their viability, proliferation, and differentiation potential.
Why 3D cultivation?
The concept of 3D cell cultures is not entirely new, explains Dr Widera; in fact, methods for cultivating cells in three dimensions have been around since the 1970s. However, the processes for 3D cultivation were traditionally cumbersome, expensive, and time-consuming. But in recent years, the interest in 3D cell cultures has become increasingly scrutinised, with several publications looking into the field, which continues to grow.
When you look at the benefits of 3D cultivation compared to its 2D predecessor, it's not hard to see why there is so much interest in it. Traditional 2D cell culture artificially affects the polarity of stem cells when you take them outside their biological niche. In any case, proliferation in 3D is far more beneficial to the integrity of stem cells.
The traditional way to cultivate stem cells in three dimensions has been to create spheroid structures of neural cells, self-generated clusters referred to as neurospheres. Alternatively, stem cells are cultivated on top of 3D beads. However, these are only semi-3D models and not the subject of Dr Widera's discussion. Rather, Dr Widera and his team are focused on systems where cells are cultured in 3D matrices of hydrogel scaffolds.
Case study I: Fibrin and Neural Crest Stem Cells
The first case study that Dr Widera took us through was research conducted by his former PhD candidate Dr Johannes Greiner. Dr Greiner looked at the cultivation of Neural Crest derived Stem Cells (NCSCs) in blood plasma-derived fibrin.
Fibrin derived from human donated blood plasma can develop networks of nano-fibres with a diameter of 40-50 nm. This network creates a healthy scaffold for cultivation, in which NCSCs are easily embedded. They grow in all three directions throughout the gel, which positively affects cultivation.
Firstly, in comparison with previous methods of 3D cultivation, such as neurospheres, the speed at which the cells cultivate is significantly increased. Dr Widera explains that the neurosphere population doubling time is around 150-170 hours. However, the doubling time using the fibrin scaffold is much more cost-effective at 24 hours, which is critical for clinical applications.
Furthermore, this kind of cultivation did not harm the cells' clonal efficiency or differentiation potential. Dr Widera added that the post-3D NCSCs are still multipotent, explaining that they could potentially specialise into bone, cartilage, fat, muscle, and even functioning nerve cells.
Case study II: Nanofibrillar Cellulose and Mesenchymal Stem Cells
Dr Jonathan Sheard and Dr Ioannis Azoidis conducted the second case study that Dr Widera discussed. The study researched the potential for cultivating Mesenchymal Stem Cells (MSCs) in Nanofibrillar Cellulose (NFC). MSCs can be isolated from various sources, including tissues from adult humans and pre- and postnatal tissues such as amniotic fluid, umbilical cord blood, or placenta tissues. These stem cells can differentiate into at least three specialised cell types, including cartilage, fat, and bone cells.
The results saw the cells interacting promisingly with the hydrogel scaffold; both the viability and the cell numbers were comparable to 2D cultivation at 0.2% concentration of NFC. Other positive outcomes from this experiment included the fact that the scientists could retrieve many cells from the NFC, a process that is difficult to do in other 3D models like Alginate.
Although there was some success with this experiment, “there was a catch”, said Dr Widera. Due to the large amounts of visible light that the NFC scaffold absorbs, this makes microscopy unfeasible. The team's solution to this was simple: anionic Nanofibrillar Cellulose (aNFC) has many of the same properties as regular NFC. Still, it absorbs far less light, making it ideal for use in a revised experiment. The MSCs favourably colonised the aNFC scaffold without a decrease in viability. Indeed, they even found that the aNFC increased the viability of the cultivation when compared to 2D. Along with this, the MSCs still thoroughly differentiated across the 3D scaffold.
The only thing left to try, said Dr Widera, was to combine the 3D cultivation with electrical stimulation, which was known to increase the level of osteogenic differentiation in MSCs. Hence, the team were interested to see if the same effect was on the aNFC scaffold. Increased levels of osteogenic differentiation were indeed observed, in “extremely high levels”, Dr Widera proudly noted.
Case study III: Blends of Synthetic and Natural Polymers with MSCs
Dr Donata Iandolo conducted the final case study that Dr Widera discussed. Using MSCs again as the cultured stem cells, Dr Iandolo worked with electrically conductive PEDOT: PSS polymers. These polymers are formed into various shapes using a freeze-drying process, making them valuable as scaffolds. The artificial PEDOT: PSS polymers were then combined with natural hydrogels, namely collagen types 1 and 3, creating a scaffold with a desirable distribution of fibres and pores.
Results showed the MSCs interacting well with the scaffold; they migrated, proliferated, and ultimately differentiated vehemently in three dimensions. The cells maintained their levels of viability, while rates of differentiation were higher than the controls. As with case study II, the natural and synthetic scaffold was then combined with electrical stimulation, which increased levels of osteogenic differentiation as before.
Conclusions
These three new models for proliferating stem cells in three dimensions indeed show great promise in cutting down the exorbitant time, effort, and money it has been associated with. Furthermore, the evidence suggests that this kind of proliferation can increase the cultured stem cells' viability, sum total, and differentiation potential. Therefore, these case studies are the beginning of better ways of manufacturing stem cells, which could be eminently game-changing for clinical applications.
Sign up for our Cell series content newsletter for the latest on 3D cell culture, therapeutic applications, and all things cell. You can also share, learn, and network with the experts in the field by joining us at our upcoming Cell UK conference later this year.