Improving Control Strategies for Bioprocessing & Cell Culture
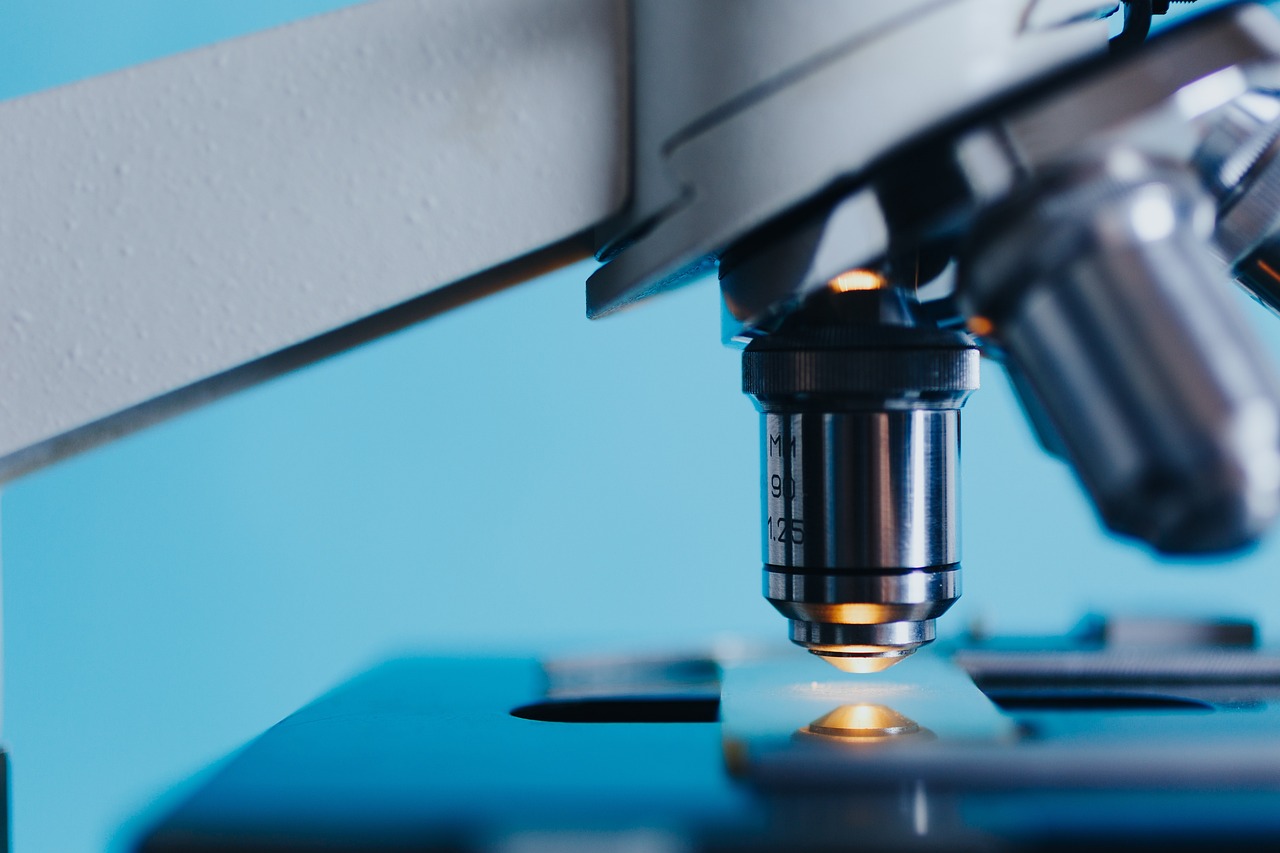
As the biopharmaceutical industry continues to innovate at an astonishing pace, the role of cell culture process control strategies becomes ever more critical. Advanced bioprocessing techniques are essential for the production of a wide range of bioproducts, from vaccines to monoclonal antibodies. Control strategies for bioprocessing and cell culture are crucial because they ensure the consistent quality and safety of biological products like drugs and vaccines. These strategies allow for the optimization of yields, which is vital for cost-effectiveness and commercial viability. They help maintain stable process conditions, which is essential in meeting strict regulatory standards and adapting to changes during the culture's growth. They're also the key to scaling up processes from the lab to industrial production without compromising product integrity or efficiency.
Advanced Process Monitoring and Feedback Control
Advanced process monitoring and feedback control are at the forefront of improving cell culture process production and robustness. The implementation of metabolic control strategies, especially in mammalian fed-batch cell cultures, aims to minimize lactic acid accumulation - a common challenge that can impede cell growth and productivity. By optimizing nutrient feed and environmental parameters, these control measures enhance process stability and product yield. A notable example is the use of online pH and dissolved oxygen sensors to adjust feeding rates in real-time, thus maintaining metabolic rates within desired ranges.
Environmental Control Strategies in Bioreactors
The control of environmental conditions within a bioreactor, such as pH, temperature, and dissolved gases, is crucial for the production of sensitive biologics like monoclonal antibodies. PID control is a critical technique used in bioreactors to maintain specific environmental conditions essential for biological processes. It operates on three distinct principles:
1. Proportional control
Proportional control adjusts the output in a manner directly proportional to the error, which is the difference between a setpoint and the actual condition. This immediate response helps to quickly reduce the error but must be carefully set to avoid excessive overshooting past the setpoin
2. Integral control
Integral control takes into account the accumulation of past errors, providing a corrective action that intensifies over time until the error is eliminated. This helps to overcome any persistent discrepancies that proportional control alone cannot handle, ensuring that the process reaches the exact setpoint without a residual steady-state error.
3. Derivative control
Derivative control looks at the rate of change of the error, essentially predicting future behavior and applying a controlling force that slows the response as it nears the setpoint, thus preventing the system from reacting too strongly and overshooting, which enhances stability. In practice, a PID controller in a bioreactor continuously monitors variables like pH, temperature, or oxygen levels, comparing them with desired values and dynamically adjusting inputs to stabilize these conditions. The tuning process is vital to balance these controls to prevent excessive correction or sluggish response.
While PID controllers are well-suited to processes with relatively predictable and stable responses, they might not always be adequate for the dynamic environment of a bioreactor where biological reactions can introduce complex variables. In such cases, more sophisticated methods like Model Predictive Control (MPC) are often employed to manage the bioreactor's environment.
Mathematical Modeling of Cell Culture Processes
One of the advanced methods used to manage this control is called Model Predictive Control (MPC). MPC works by using a mathematical process model that can predict the future state of the bioreactor's environment. It takes into account the current conditions as well as historical data to make these predictions. The system then uses these predictions to make adjustments in real-time to maintain the desired conditions. For instance, if the model predicts that the pH level will rise beyond the optimal range, the control system can adjust the addition of acids or bases to maintain the pH at the desired level. The same goes for temperature and dissolved gases like oxygen or carbon dioxide.
This preemptive approach to adjustment means that the system is not merely reacting to changes but is actively anticipating and mitigating them before they occur. This leads to a more stable environment, which is essential for the consistent production of high-quality biologics. The MPC strategy is particularly useful because it can handle multiple inputs and outputs simultaneously, meaning it can control several environmental factors at once. It is also adaptable to changes and can learn from new data, which is especially important in bioprocessing, where living organisms can have unpredictable responses to their environment. Overall, the use of MPC in controlling bioreactor environments represents a significant advancement in bioprocessing technology, enabling more efficient, reliable, and high-quality production of biologic medications.
Process Analytical Technology (PAT) Tools
Process Analytical Technology (PAT) tools are systems and methods implemented in the manufacturing process to monitor and control it in real-time. These tools are designed to measure critical quality and performance attributes of raw and in-process materials and processes with the goal of ensuring the quality of the final product. The use of PAT is a shift from traditional quality testing of end products to a more proactive approach that focuses on understanding and controlling the manufacturing process itself.
In bioprocessing and cell culture, PAT tools are used to assess the condition of the culture, such as pH, temperature, nutrient levels, and product concentration, providing immediate data that can be used to adjust the process as needed. This real-time monitoring allows for immediate intervention and optimization, reducing waste, increasing efficiency, and ensuring that the bioproducts meet the desired standards of purity, potency, and safety.
The implementation of PAT is part of a broader regulatory initiative to incorporate quality by design (QbD) in pharmaceutical manufacturing. This approach emphasizes the importance of designing processes that inherently have a high probability of producing high-quality products. By using PAT tools, manufacturers can better understand their processes, leading to fewer product failures and recalls, and ultimately to a more efficient and predictable manufacturing process.
Sartorius has been noted for its advancements in single-use technologies, which have significantly changed biopharmaceutical production processes. These single-use sensors are highlighted for their robustness and reliability, which enhance the benefits of single-use processing concepts and allow for the effective application of PAT??.
In conclusion, the continuous evolution of cell culture process control strategies is essential for maintaining the competitiveness and effectiveness of bioprocessing operations. By integrating advanced monitoring systems, sophisticated control strategies, and predictive models, biotech and pharmaceutical companies can achieve higher yields, improved product quality, and enhanced process robustness.
Get your weekly dose of industry news and announcements here, or head over to our Cell portal to catch up with the latest advances in stem cell development and manufacturing. If you'd like to learn more about our upcoming Cell UK conference, visit our event website to download an agenda or register your interest.